Why insist on novelty for decarbonized commodities?
Are we looking for new love in all the wrong places?
Decarbonization isn’t just about a shift in energy sources. Judging by all the new projects out there in academia, industry and the startup world, decarbonization also portends enormous changes in how we use energy to make things.
The old way was thermochemical. Since fossil carbon is both a good source of heat and a good chemical reducing agent, our legacy chemical processes often resemble explosions that have been cleverly frozen at various stages to make the products we want. Cement, steel, plastic, fertilizer[1]—and so much else. Think of the dirty roar of a giant steam reformer, chemical cracker, or blast furnace.
The new ways seem to be either electrochemical or biological. Those are the most obvious ways to use green electricity or agricultural feedstocks to make the products we get from fossil energy now. Think of the quiet power of an electrolyzer, or the bubbling of a fermentation vat[2].
So while the explicit assumption of a net-zero materials economy is that baseload green energy will become widely available and affordable, an implicit assumption is that these very different methods of materials production can someday mature as drop-in replacements for the processes we run now.
But is that right? There are reasons to doubt that electrochemical or biological processes can sub in for thermochemistry so easily. All else being equal, my priors say a thermochemical process will beat an electrochemical one at scale; a reaction in a volume is effectively “3D” while one on an electrode surface is “2D”—hence the thermochemical process has a power law advantage when it comes to reaction rate per capital expense. I have similar skepticism about bioprocess scaling. Biology happens in water, at mild temperatures, at neutral pH, while chemical processes can happen at extremes of temperature, pressure, equilibrium constant—whatever it takes to make the product cheaply and quickly. Of course thermochemistry will win.
But those are just prejudices! To interrogate them, let’s look at some data on the balance between thermochemical, electrochemical, and biological production methods for the materials we use today.

Electrochemistry vs thermochemistry
To get specific, my prior was that all else being equal a thermochemical process will win on scale (3D vs 2Dequipment), but that electrochemistry can win in specific cases where there are coproducts (like chlorine production) or very reducing potentials (like aluminum production)[3].
My colleague Aravindh and I decided the best way to stress-test that assumption was to make a map of all major inorganic chemicals, sorted by the energy of the reaction (in voltage,energy per electron) and value per energy (i.e. what fraction of the value of the commodity is its embodied energy). The dot sizes are market volumes in tons per year on a log scale.
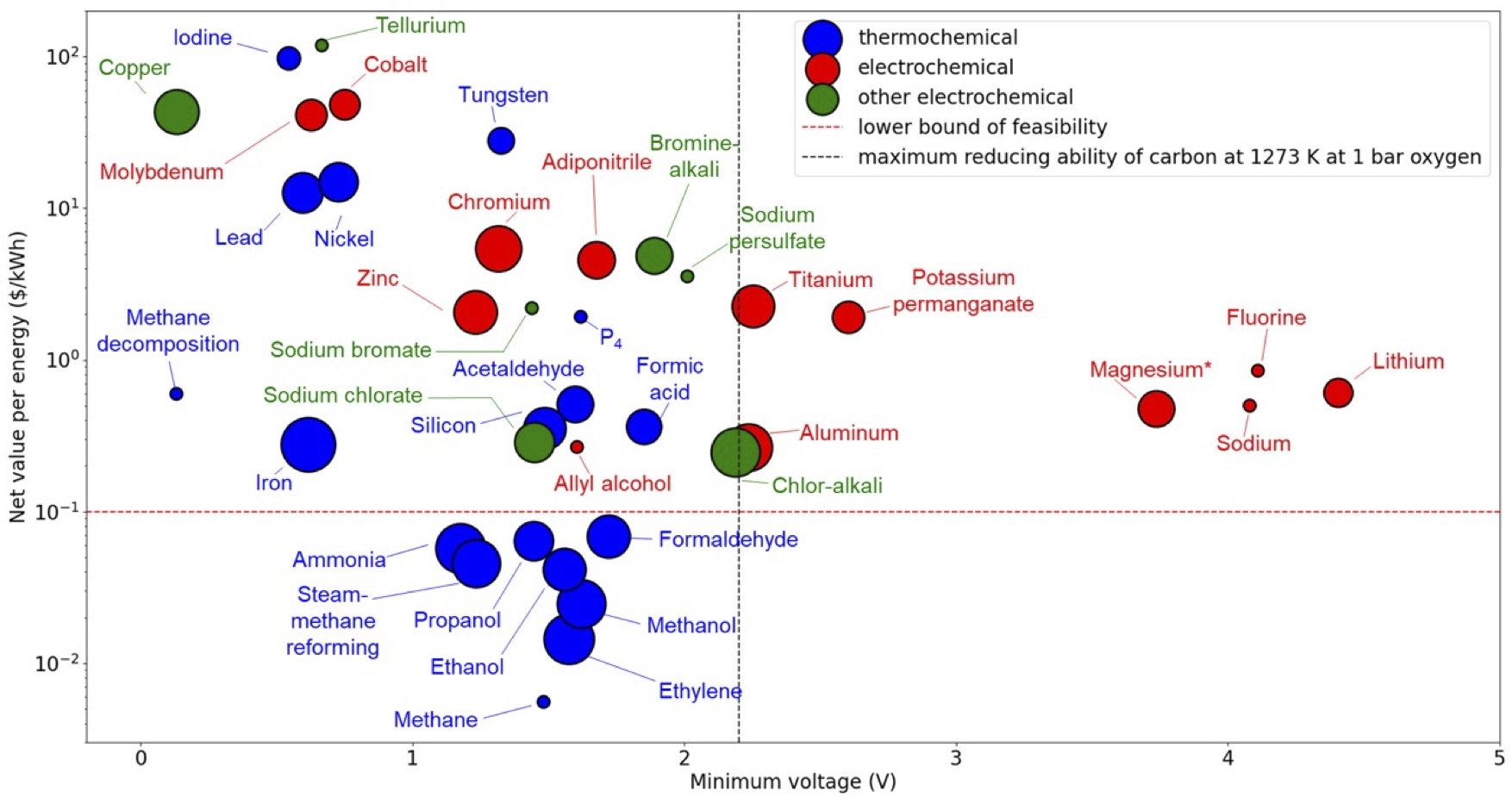
We dropped a horizontal line on the plot to denote the lower bound of feasibility for electrification based on electricity prices. We also dropped a vertical line to show the limit of what you can reduce with carbon up to 1000ºC. That gives a plot with four quadrants.
In the top right quadrant, electrochemistry is basically obligatory. You can’t reach those potentials with carbon as a reducing agent, and every single commodity there is already produced electrochemically. In this region, the energy transition is ready to go. We’re just waiting on the clean electricity.
In the bottom left quadrant,fossil-powered thermo chemistry is universal for energy cost reasons.Electricity is not cheap enough, and electrochemical reactors are at additional disadvantage from scaling. These processes will be very hard to decarbonize without substantial subsidy.
The top left quadrant is where it gets more interesting. Both thermochemistry and electrochemistry coexist, but nearly every extant electrochemical example brings in a secondary advantage like purification (e.g. Cu), surface sensitivity (e.g. galvanization), a veryoxidizing potential (persulfate), or valuable coproducts (chlor-alkali). Those are the green points. Basically, electrochemistry needs to serve as more than a mere method of reduction to compete in this region[1].
Biology vs. chemistry
My prior here was that biology wins at making complex compounds, chemistry wins at making simple things cheaply. This view came from looking at how things like the amino acids are produced; where chirality doesn’t matter (glycine and methionine) they’re made chemically, where it does (all the rest) they’re made either biologically or semi-biologically with immobilized enzymes.
What happens when you shakeout that prejudice by looking at the whole chemical industry?
Here are the top 100 organic chemicals by market value:
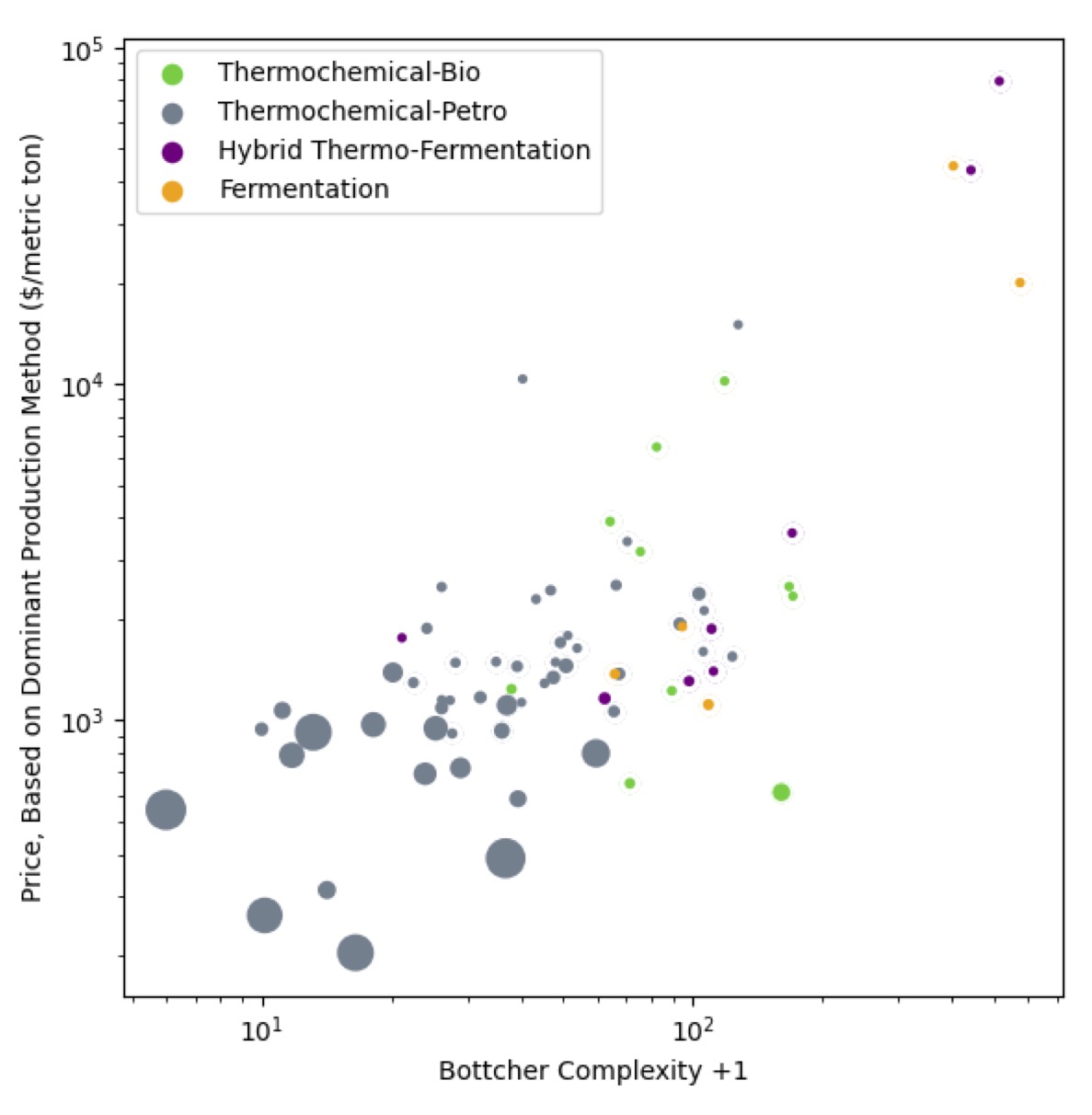
[1]One thing that would blow up this whole worldview: cheap geologic H2.
Planet earth tends to be acidic and oxidizing on theoutside, basic and reducing on the inside. Those potential gradients have forall human history been basically unreachable to us, blocked by many miles ofrock.
But if the universe’s most mobile molecule is indeedcapable of connecting those two worlds, all of this would change—that energywould suddenly be accessibly.
I’ve heard claims of large scale geologic H2for $0.80/kg with <0.5kg CO2/kg H2. That’d changeeverything. The story of decarbonization would no longer be aboutelectrification. H2 after all is a chemical reducing agent.Everything on the left half of the plot would be made with H2. Suddenlyour world would flip and those would be the easy to decarbonizecommodities.
Footnotes
[1] Vaclav Smil, https://time.com/6175734/reliance-on-fossil-fuels/
[2] It’s hard to overstate how widespread this is. When researchers and climate entrepreneurs want to replace a polluting inorganic chemical, the tool they reach for is electrochemistry. Electrochemical cement, steel, ammonia, hydrogen etc. And when they want to decarbonize an organic chemical, the tool they reach for first is nearly always synthetic biology. Bio-ethanol, bio-plastics, biofuels etc. Projects of this nature are so widespread that you’d think there were a law on the books.
[3] Fossil carbon provides a means of reduction, which combined with our planet’s oxidizing atmosphere makes for a convenient source of energy. Electrochemistry always couples both an oxidation and a reduction reaction—ideally, you can find value in both.