The future is made of energy
A megatrend way bigger than decarbonization is probably the key to abundance and sustainability both.
Here’s a big secular trend that you’ve probably thought about, but maybe not as much as you should: over time our world is getting to be made more out of energy, and relatively less out of matter.
In the 1960s Coke came in glass bottles. Fragile melted sand. Today Coke comes in aluminum cans – a material that takes tremendously more energy to produce, but ends up being cleaner and cheaper and 40x lighter than glass holding the same amount of liquid[1].
The same transformation has happened in almost every corner of our material lives. It has been happening for thousands of years and in every material category. Over time people have switched from mud to brick to cement, from wood to plastics to fiberglass to carbon fiber, from copper to bronze to steel to titanium, from leather to cotton to nylon, from smallholder to factory farms, from heaps of coal to thin wafers of solar PV, from vacuum tubes to specks of semiconductor, from highways to runways to Zoom. Energy intensity increases, materials get stronger and lighter, machines pack more power into smaller spaces. A signature element of modernity is how we’ve moved from materially-centered ways of doing things to energy-centered ways of doing things.
Some people call this trend ‘dematerialization’. But that’s misleading. The world today may be made relatively less of stuff, but for various growth and demographic and Jevons reasons overall there’s way more stuff than ever. So I think it’s best to think of it as our world being made more and more out of energy.
A world made more and more of energy

One reason for this trend is that investing more energy gets us lighter and tougher materials[2]:
.png)
Another piece of the trend is that more energy often gives us the flexibility to build what we want out of more plentiful stuff[3].
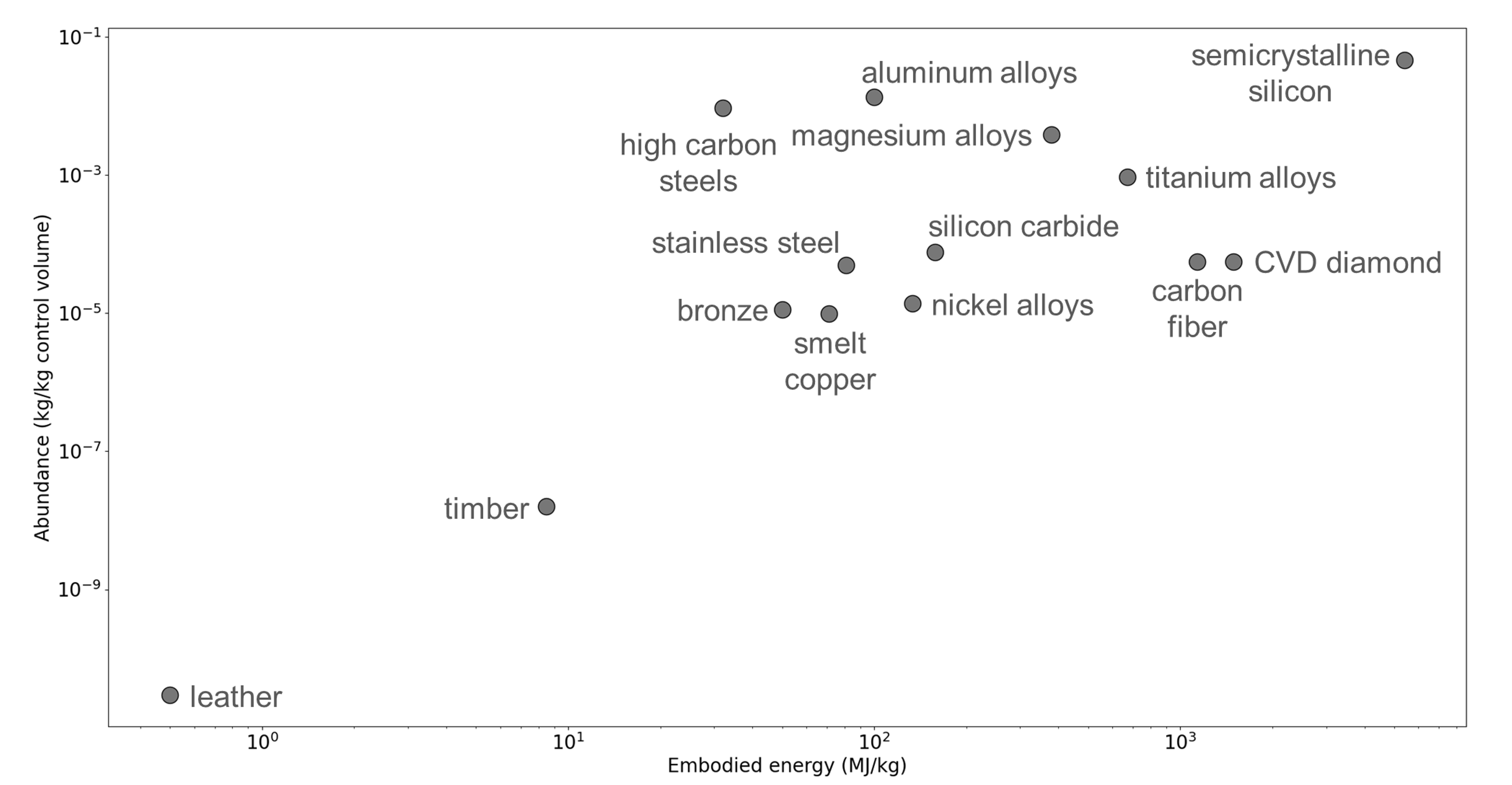
Smelting, refining, structuring, purifying—almost everything we can do to give a material new properties takes energy. So it shouldn't be a surprise that our highest performing materials have become more energy intense over time[4].
...and relatively less of "stuff"
Lighter and stronger materials are an easy-to-see example of dematerialization. But "more energy--> less stuff" goes way past the built environment. I’ll describe how I see it acting in a few major sectors—if you look around you’ll see it elsewhere too[5].
Food
You can probably divide the history of food production into four or five phases:
1. Hunting and gathering. Huge land requirement for each person.
2. Early agriculture. Much less materially intensive (less land per person) but more energetically intensive (human labor, tilling, collecting waste as fertilizer)
3. Semi-mechanized agriculture before Haber-Bosch. Millions of tons of collected or mined fertilizer (e.g. guano or Chilean nitrates) decrease land intensity, increase energy use.
4. Modern agriculture. Fertilizer comes directly from copious (~25GJ/t) energy and abundant air. It takes ~6x less land and ~100x more energy to make a calorie of food than it did in 1900. But agriculture is still materially wasteful—severalfold more biomass is produced than ends up on our plates, and half the habitable land on the planet is in some way used for food production. Reading the trend, we should energize it further if we can[6].
5. The Savor era? Some food can be made directly from energy and air….

Energy
Energy sources themselves have dematerialized, from biomass to fossil to renewables to (potentially) nuclear:
• Coal represented an enormous dematerialization from the previous era of biomass power. Contrast coal or petcoke and the era of charcoal-based steelmaking which required vastly more material input and annihilated forests in much of Europe.
• Solar PV cells are made of the second most abundant material in the earth’s crust, processed with copious energy to yield an energy converter that’s 2-3 orders of magnitude more mass-efficient than coal. As multi-junction PV rolls out, expect another 1/3rd or so reduction in the total material requirement and another doubling in the embodied energy for solar power.
• Nuclear fission of enriched uranium provides another 2-3 orders of magnitude more energy per mass than solar over a 20 year lifetime. Nuclear fusion if it’s ever useful (🤷♂️) would compound that by using a fuel that’s virtually limitless in the oceans[7].
Getting around
Muscle-powered transport on grazing animals to combustion of fossil fuels to electrification. Roads (1/3 of all materials used in our world) to flight to Zoom. [8]
Semiconductors and computation
As logic elements have gotten smaller, computation is more energy passing through smaller and smaller bits of material. From vacuum tubes to FinFETs, our computers have gone down in material intensity and up in energy intensity with every Moore’s law doubling. And that’s happening as the semi substrate itself is also getting more energy intense--as we move to higher band gaps, we’re driven to lighter atoms which take more energy to purify.
Et cetera
You see it in other sectors too. Modern economies reorienting from goods to services. Cards or crypto over cash, life moving online, "Everything is computer". And of course the central meme of our time, AI augmenting then replacing humanity.
Frame it broadly enough and it's almost tautological. You can almost define invention as applying energy to make things nicer[9]. That makes the trend feel inevitable—as long as we move forward technologically, of course everything will come to be made more and more out of energy.
The opposing view
Strangely you don’t encounter this “the future is always made more and more of energy” view out there much. In fact, the mainstream perspective is basically the opposite. More like “the future should take less and less energy”.

Many people observe that energy use skyrockets as a country industrializes, but then flatlines or declines afterwards. Nations switch from mud and straw to cement and steel exactly once. Same when fields start getting fertilized, stuff starts getting wrapped in plastic, roads get paved and people get cars. Once that happens, material needs are mostly met. From that point, incentives point to efficiency and cleanliness—making the same stuff with less and less energy[10].
This view manifests as a kind of “End of History” attitude when it comes to the future of materials: just a few materials underpin the whole modern way of life, they are so essential and the way we make them has gotten so efficient over time that it’s hard to imagine we’ll ever build our world out of anything else. As Vaclav Smil writes, it’s Still the Iron Age—and may always be.

“Less and less” is probably a somewhat adaptive attitude. Here in 2025 we sit in the middle of a multi-century transition from a powerful but toxic energy source to new sources that will probably prove to be limitless but until recently have seemed weak and expensive. You get why people might not see “more and more energy” as the inevitable future.
But obviously I think it’s a mistake to look at things this way. Using more energy is almost definitionally how we unlock new capabilities, especially where materials are concerned. It seems clear that basically all the good things we associate with modernity came first by increasing embodied energy (often substituting new materials), and only later by whittling it back down (increasing efficiency and minimizing externalities)[11].
That makes me a conscientious objector to some parts of the decarbonization agenda. The “less and less” attitude has everyone in materials R&D focused on greening the end-of-history tech (steel, cement, plastic, fertilizer) or even moving backwards (biofuels, cell-based wood, low-yield organic ag etc.). It’s out of touch with the longer contours of history and the new materials and capabilities that people will actually want. It's even out of touch with what has most helped the earth. The biggest environmental triumphs of our time—obscured as they are beneath the backdrop of constant population growth—came by spending more energy, not less[12].
Back in Cleantech 1.0, all the subsidy programs aimed at reinventing what we already had were failures (e.g. biofuels, solar thermal, CNG cars). Others that targeted categorically new and somewhat dematerialized energy (solar PV, big wind, some Li-ion) were derided at the time but look like victories now. I have no doubt a post-mortem on Cleantech 2.0 in a few years will show the same trend.
So I like stuff that follows the "more and more energy" trend instead. New alloys and ways of making them, new ceramics and composites, new ways moving around or making food etc[13]. Obviously all of that is predicated on the view that we'll eventually have copious cheap energy, still a big "if". But that's prerequisite for the 'electrify everything' worldview anyway[14].
That brings up the concern I mentioned earlier that all the free money for decarbonization might hold us back from the future we actually want. Programs like the IRA look progressive, but outside of removing one acid gas they mostly subsidize and entrench the end-of-history technologies we've had all our lives. E.g. if steel is the world’s main structural material in today’s economic equilibrium, but with innovation and an unsubsidized energy transition the global equilibrium would show Al, Mg, Ti as better-performing and lower-carbon alternatives in the future[15], then subsidies for green steel make that future harder to achieve. There’s a risk that all the government and VC handouts for decarbonizing the status quo could lock us in the material economy of the 1950s for a long time.
Having it both ways
Older sci-fi often had the feeling of “freedom through expansion”. Ever-growth, the expanding light of consciousness into the infinite frontier of space. You’ve probably noticed that newer sci-fi has felt different—dystopia, inequity, escape from subjugation or constraint.
Real science and technology have shifted the same way. The old goals were abundant energy and amazing materials, a world where human capabilities were meaningfully increased. For all my adult life, the new goals have been decarbonization, degrowth, atonement. Reinventing the old stuff without harmful externalities for the long end of history.
In the last year or so a piece of the old strain is back in ascendance. More and more new companies are pursuing a master morality of technology development. Decarbonized commodity startups are rebranding as critical mineral companies, climate VCs are pivoting to “abundance”. Some of this is protective coloration for entrepreneurs who still believe in climate and sustainability goals. But the tension is clear: one set of technologists wants immortality and Mars rockets and supersonic driverless planes, another still wants carbon-free cement and direct air capture.
What those two visions for the future have in common is that they both seem pretty fake without incredible new materials and copious new energy. The trick to having it both ways is the same as it has always been—more energy used to dematerialize our lives, our transport, our food, and to make lighter, stronger, better materials.
At Orca recently my favorite projects have all been about making the world more of energy, and they’re all still decarbonization-relevant. Light metals, composites, better purity, high-spec ceramics, parts engineered down to the μm scale for physical or electromagnetic properties. Stuff that will help things spin faster and hotter, last longer, use less material etc. Our new project Light Materials is almost the apotheosis of this vision—quickly and cheaply fabricating ultra-performant materials in beams of pure energy. It’s not tech that lets you peddle indulgences for supposedly mitigating GTs of CO2 this decade, but it feels good working on stuff that blows peoples minds and that can plausibly lead us to a future that’s both less of what we don’t want and more of what we do[16].
Footnotes
[1] I chose this example since it’s so familiar and extreme but the whole situation with beverage containers is pretty confusing and probably a lesson in how engineering requirements compete with other requirements in the real world. Cans take 10x the energy to make per kg but being 40x lighter are about 4x less energy overall than glass bottles. Since they’re also more recycleable they up being about 5-8x better on a life cycle CO2 basis. But glass bottles are still all over! That physicochemically identical liquids could be packaged in glass, aluminum, laminated paper, or multiple different kinds of plastic, and that those solutions would be basically at equilibrium on the market for packaging is pretty amazing.
[2] It’s important to caveat that if you size the dots in these first few figures by production volume, the most energy-intensive materials would look like a modern sideshow, not an inevitable future.
If you’re inclined to get captious with the trends here, take it this way: the point is that energy is and has always been our main lever in exploring the parameter space of new materials, to go farther through the search space we need more energy (as reduction, processing, purification, structuring) so the cool new things we come up with are almost always gonna take more energy than what we use already, and people shouldn’t be afraid of that
[3] All this is obviously ongoing. If we’re going to make a structural material out of carbon, today it could be solid wood from virgin trees (high material intensity, low energy intensity), particleboard made from sawdust and natural gas based glue (lower material intensity, medium energy intensity) or carbon fiber made directly from atmospheric CO2 (near zero material intensity, tremendous energy intensity).
[4] What are the limits to a trend like this? You might look at the electrochemical series and point out that past Al or Mg there isn’t much more energy you can invest in a metal. For allotropes of carbon, you can go to carbon fiber or ultrapure diamond but the theoretical energy input can’t get that all that high. Maybe that’s why civilization seems to have plateaued at the materials we have today.
But I don’t think we’re anywhere near the limit. Our colleague George Hansel points out that if you split the energy intensity of materials into a Gibbs free energy component (enthalpy plus purification, practically) and an additional entropic component that we add by doing computation and structuring the material there’s a lot further to go. Probably 100x with high-entropy materials. And then when you consider structured materials things can really blow up. The Nvidia GB200 runs around 5x10-15 joules per bit of entropy, a factor of a million off the Landauer limit at room temperature. So while we may someday hit marginal gains in adding more free energy to a bulk material, there’s plenty of room to run in highly-structured materials.
[5] Some examples that cut across categories:
The nickel-based blades in a 1960s turbofan took more energy to smelt and age than an entire WWII aviation piston block engine, yet that swap cut trans-Atlantic fuel per passenger mile in half
GaN epitaxy means manufacturing an LED lightbulb takes 7x as much energy as manufacturing an incandescent lightbulb, but it lasts vastly longer, reducing overall material requirements ~5x.
In 1909 Fritz Haber fired a reactor that guzzled 25GJ of heat per tonne of ammonia—100x the energy of shovelling compost for fertilizer – but within two decades crop yields had doubled on the same acreage and any other source of nitrogen fertilizer was uneconomic.
When the first Bessemer converter blew air through molten iron in 1856 it consumed more fuel than any puddling furnace, yet by 1875 95% of the world’s rails had switched from wrought iron to steel and the age of skyscrapers began.
[6] Depending on how you draw the control volume, the energy subsidy can look even more severe. You can find clams that world agriculture takes in more calories of energy as {oil, gas, electricity…} than we end up eating in the form of food calories. Certainly that pencils if you zoom out to a net GHG standpoint. World ag is ~ 5% of direct CO2 emissions (ex land use, non-CO2 GHGs etc.). Since all human exhalation is also ~6% of world anthropogenic CO2, that implies that around the same amount of fossil C is burned in the food production process as we eventually get as food calories.
[7] The oceans aren't just for fusion. They contain enough economically-extractable U to power humanity for a thousand years.
[8] If we all flew everywhere on jet planes instead of driving on roads, flying would still represent a relative dematerialization from road travel. That's true even if the planes were powered by fossil hydrocarbons (mass of world road network ~= 160GT, mass of CO2 emitted if this year's world total of 50 trillion passenger kms were shifted to jets at the 90gCO2/passenger km average for jets ~= 4GT).
[9] I’ve been sloppy with energy terminology throughout this note, but this is clearly a new low. Above I’ve included in ‘energy’ a mix of free energy and entropic concepts and use them almost interchangeably. Probably best to expand the sense of "energy" to e.g. carbon fiber is a ‘high energy material’ not just from the literall process energy but because making it is capital intensive and requires multiple process steps, imperfect yields, and multiple feedstocks mixed in precise ratios.
[10] There’s a piece of the “great stagnation” meme here. Three new structural metal industries (Al, Mg, and Ti) were introduced in the half century before 1970, while energy was generally getting cheaper. No new major structural materials have been introduced since. Maybe we lost oil as the universal marginal energy currency, energy costs stopped declining, we concomitantly stopped substituting newer high-performing high-energy materials for old ones, and since materials properties are upstream of almost all device performance “where’s my flying car?” was born.
If you look at some of the "End of History" curves, the more nuanced story seems to be that energy-enabled dematerialization enhanced our capabilities until the 70s, energy-less dematerialization put us into “and I only got 140 characters” mode ever since.
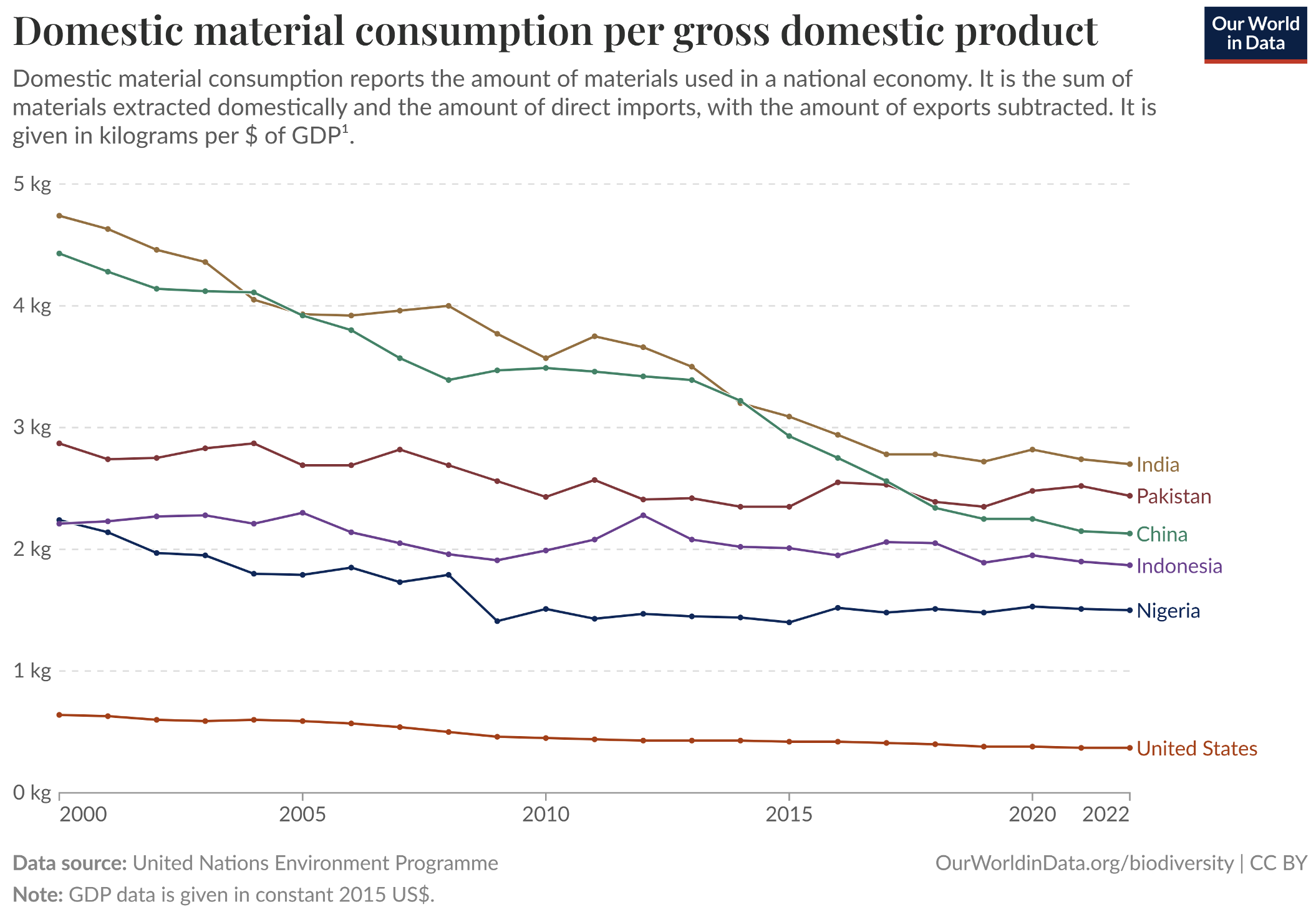
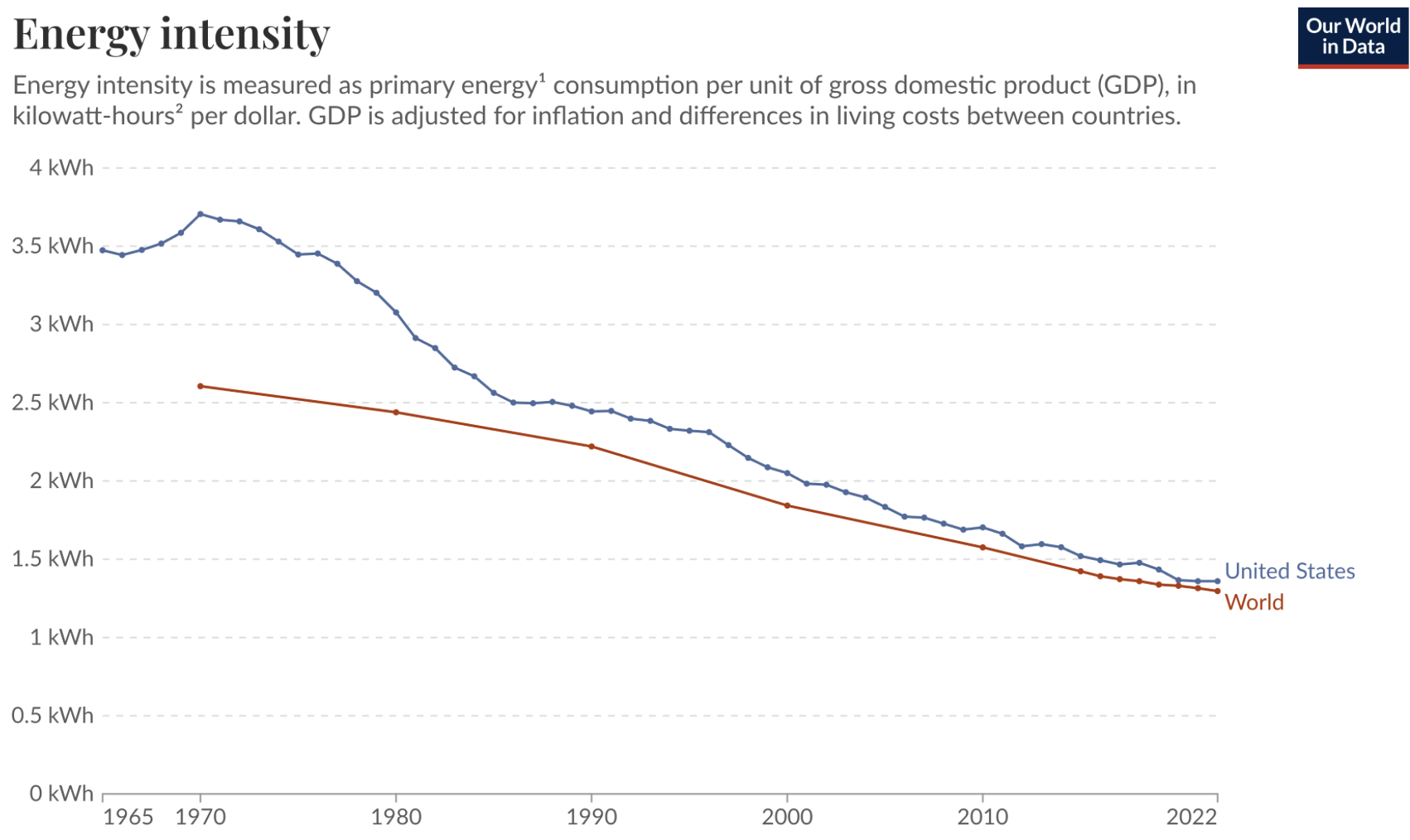
[11]There's romance in those lower-energy lifestyles of the past. Whether it’s organic 1800s agriculture or 1700s wrought iron from charcoal or 1600s animal motive power or ancient hunting and gathering — that kind of stuff can make nice wholesome affectations for rich people. You're gonna see trust fund kids going hunting and eating organic and making pottery and riding horses forever. But obviously it would but be globally ruinous if we try to roll back to any of that for all 8 billion of us.
[12] To name just two of many examples, 1) the reduction in agricultural land use via energy intense farming practices and fertilizers (without which the forests of the earth would have to be entirely cleared in order to feed 10^10 people) and 2) fuel desulfurization and catalytic conversion (in two fell strokes basically defeating air pollution in the industrialized world). Both examples of a “more energy” approach solving environmental problems that in some ways might be considered more serious than climate change.
[13] Not to mention dark-horse decarbonizers like Zoom or Ozempic.
[14] You might be inclined to point out that decarbonizing the commodities we have is consistent with the long term "more energy, less stuff" trend. After all, use green electricity to make steel or cement and you're replacing huge masses of fossil fuel with clean electrons or abundant water and CO2. But the real unstoppable trend is giving people new capabilities they actually want. That's the key to getting on the right side of Austin's clean energy barbell.
[15] This is a handy example and nicely riffs off Sam Faucher’s recent paper. But it’s probably not realistic that Al, Mg, Ti, or carbon fiber could replace steel wholesale, even if we had much cheaper energy. Material substitution is tricky and a lot of secondary design choices depend on the materials we already use. In these discussions our colleague Patrick will also point out that so many steel has properties that none of the “fancy materials” can match:
“We get excited about light metals because they look good on Ashby plots where the figure of merit is E1/2/ρ [root Young’s modulus over density]. But this is only the right figure of merit for a beam whose size is unconstrained. Often the size is constrained. What then? When stiffness per volume matters, steel wins handily. Moreover, when the object is purely under tension like a rope, the figure of merit becomes E/ρ, and using this figure of merit no metal looks that much better than the next! Similar comments for strength-limited designs. So why would we think that huge swaths of steel could or should be replaced by light metals? The single biggest use of steel is for rebar, which is about tensile loads and the weight consideration isn’t that important. Properties like CTE [coefficient of thermal expansion] match, stiffness and strength per cost, ductility, and weldability make steel the obvious choice. Many cars still rely on steel even though weight is at a premium, at least in part because steel can be formed easier and into structures with thinner walls. I think it’s best to view all these metals (and certainly carbon fiber, which is so different that it almost doesn’t belong in this discussion) as very different materials that could be the right one in any given application for a whole host of reasons, not as inevitable replacements for steel.”
[16] I’ve been pretty one-sided in this note in order to support a perspective I think is undervalued. Obviously a lot is elided. Clearly the end-of-history view of materials is mostly the world we live in and Vaclav is correct in pointing out that we're still in the iron age. But we're in technology development and if you can't you can't squint and see a positive vision of the future you're probably missing basic table stakes for making new stuff at all.
References
The main reference here was Vaclav Smil's Materials and Dematerialization. It's the Vaclav book that has made by far the greatest impression on me.
The other Vaclav books are helpful but can be downers. The books on energy transitions, agriculture and iron show how the world has converged on a set of materials that underpin almost every aspect of our existence, and how they're made at such a scale that it's hard to imagine them ever changing. But Materials and Dematerialization is almost the opposite. It shows you step by step how more energy input has let us hop to better and better materials over time. It's definitely his most hopeful book.
1. Ashby, M. F. (2013). Materials and the Environment: Eco-Informed Material Choice (2nd ed.). Butterworth-Heinemann – fig. 1.1; app. A.10.
2. McKerrell, H., & Tylecote, R. F. (1972). “The working of copper–arsenic alloys in the Early Bronze Age and the effect on the determination of provenance.” Proceedings of the Prehistoric Society, 38, 209-218. https://doi.org/10.1017/S0079497X00012111
3. Patel, S. J. (2006). “A century of discoveries, inventors, and new nickel alloys.” JOM, 58(9), 18-20. https://doi.org/10.1007/s11837-006-0076-y
4. Runge, J. M. (2018). The Metallurgy of Anodizing Aluminum: Connecting Science to Practice. Springer. https://doi.org/10.1007/978-3-319-72177-4
5. Warren, J. A., Riddle, M. E., Graziano, D. J., Das, S., Upadhyayula, V. K. K., Masanet, E., & Cresko, J. (2015). “Energy impacts of wide band-gap semiconductors in U.S. light-duty electric vehicle fleet.” Environmental Science & Technology, 49(17), 10294-10302. https://doi.org/10.1021/acs.est.5b01627
6. Zhai, P., & Williams, E. (2010). “Dynamic hybrid life-cycle assessment of energy and carbon of multicrystalline silicon photovoltaic systems.” Environmental Science & Technology, 44(20), 7950-7955. https://doi.org/10.1021/es1026695
7. Zhdanov, V., Sokolova, M., Smirnov, P., Andrzejewski, Ł., Bondareva, J., & Evlashin, S. (2021). “A comparative analysis of energy and water consumption of mined versus synthetic diamonds.” Energies, 14(21), 7062. https://doi.org/10.3390/en14217062
8. Muthu, S. S., Li, Y., Hu, J. Y., & Mok, P. Y. (2012). “Quantification of environmental impact and ecological sustainability for textile fibres.” Ecological Indicators, 13(1), 66-74. https://doi.org/10.1016/j.ecolind.2011.05.008
9. eStainlessSteel. (n.d.). History of stainless steel. Retrieved 22 May 2025 from https://www.estainlesssteel.com/historyofstainlesssteel.shtml
10. Science History Institute. (2023). “Magnesium: From the sea to the stars.” Retrieved 22 May 2025 from https://www.sciencehistory.org/stories/magazine/magnesium-from-the-sea-to-the-stars/
11. International Titanium Association. (n.d.). Black sand: The mineral origins of titanium. Retrieved 22 May 2025 from https://titanium.org/page/BlackSand
12. Strine Environments. (n.d.). Concrete for carbon-neutral housing – fact sheet. Retrieved 22 May 2025 from https://www.strineenvironments.com.au/factsheet-concrete-for-carbon-neutral-housing/
13. Hanson Malaysia. (n.d.). Concrete mix ratios & types. Retrieved 22 May 2025 from https://www.hanson.my/en/concrete-mix-rations-types
14. One Click LCA. (2019). Average quantities of reinforcement in concrete. Retrieved 22 May 2025 from https://oneclicklca.zendesk.com/hc/en-us/articles/360020943800-Average-Quantities-of-Reinforcement-in-Concrete
15. Housing.com. (2024). Density of concrete – how heavy is it? Retrieved 22 May 2025 from https://housing.com/news/density-of-concrete/
16. Australian Government, YourHome. (2023). Embodied energy. Retrieved 22 May 2025 from https://www.yourhome.gov.au/materials/embodied-energy
17. Architect Magazine. (2020). “A history of wood: From the Stone Age to the 21st century.” Retrieved 22 May 2025 from https://www.architectmagazine.com/technology/products/a-history-of-wood-from-the-stone-age-to-the-21st-century_o
18. California State Parks, Office of Historic Preservation. (n.d.). Embodied energy (technical bulletin). Retrieved 22 May 2025 from https://ohp.parks.ca.gov/pages/1054/files/embodied%20energy.pdf
19. Wikipedia contributors. (2025). Bronze. In Wikipedia, the free encyclopedia. Retrieved 22 May 2025 from https://en.wikipedia.org/wiki/Bronze
20. Wikipedia contributors. (2025). Muntz metal. In Wikipedia, the free encyclopedia. Retrieved 22 May 2025 from https://en.wikipedia.org/wiki/Muntz_metal
Thoughts in here originated with Menyoung Lee, Patrick Gallagher, Eli Dourado, Austin Vernon and George Hansel. Graphs were made with Aravindh Rajan.